TU researchers are testing ammonia from urine as a feed for fuel cells. The DHV-led project aims to make plants for wastewater treatment self-sufficient in energy.
A number of technologies have been developed to remove nitrogen and phosphates from sewage water as energy-efficiently as possible. The recently distinguished Anammox method developed by Professor Mark van Loosdrecht (AS) is one such technological application. DHV consultancy chose another tack: in an experimental plant near Groningen, they removed the nutrients chemically in order to optimise energy production. This DHV water innovation project was awarded the 2010 ‘Vernufteling’ award, as it saves energy and recycles phosphates at the same time.
The process involves adding magnesium hydroxide to the wastewater. The magnesium binds the phosphate and nitrate into an insoluble salt, which then precipitates. It is this salt, called ‘struvite’, which is transported from Groningen to Delft and stored in tanks for fuel tests.
“When you heat the salt, ammonia escapes,” explains Sander Tensen, who graduated on the overall system engineering. In fact, it’s a mixture of ammonia and water vapour that enters the system. When heated to over 750 ºC, ammonia will split up into its constituents: nitrogen and hydrogen. The high temperature fuel cell (solid oxide fuel cell) burns hydrogen with oxygen (from the air) while producing electrical power with an efficiency of about 50 to 60 percent. “And no nitrogen oxides or NOx’s are formed,” says project manager Dr P.V. Aravind, from the 3mE faculty’s process and energy department.
“Practice often proves to be a little more challenging,” says Tensen, as he explains that besides the ammonia, water vapour also enters the fuel cell. This not only dilutes the process but may reduce the performance of the fuel cell as well.
“The main thing is to get waste water plants self-sufficient in energy,” says Aravind. Especially in developing countries, sewage treatment is often interrupted by power cuts. Fuel cells fed with biogas from the sewage water treatment, as well as ammonia from urine, should be able to keep the plant going.
There are treasures waiting at the bottom of the deep sea. Gold, silver, copper, and manganese are all to be found in exceptionally high concentrations in the sulphide deposits created by hydrothermal vents at about 1,400 to 3,700 meters below the ocean’s surface.
Subsea mining was first proposed in J.L. Mero’s 1960 publication, ‘Mineral Resources of the Sea’, which noted the sheer limitless supply of cobalt, nickel and other materials in deposits of manganese nodules at depths of 5,000 metres. But after some initial expeditions on the seafloor, global metal prices began falling due to the weak economy of the 1980s, and hence the prospect of nodule mining was abandoned. But recent increases in demand and prices of metals have put deep-sea mining back on the agenda. This time however it’s targeted at the volcanic hydrothermal vents, or black smokers, where hot mineral-rich water springs from the ocean floor, creating towering deposits compiled from a zoo of metals and sulphur.
Front runner in deep-sea mining of Seafloor Massive Sulphide (SMS) resources is currently Nautilus Minerals (UK) with a concession for mining gold and copper at a depth of 1,600 metres off the coast of Papua-New Guinea. First production is expected in early 2013. Like Canadian company Neptune Minerals, operating near New Zealand, Nautilus will also venture into phosphate mining. Dredging expert, Professor Cees van Rhee (from the faculties of Mechanical, Maritime and Materials Engineering, and Civil Engineering and Geosciences), expects that phosphate mining, taking place nearer to shore and at a limited depth (400 metres), will be one of the first profitable maritime mining activities. Van Rhee: “The price of rock phosphate has peaked to USD $400 per tonne. It can be mined from the sea at approximately $100 per tonne.”
The dredging expert says it’s not so much a question of if deep-sea mining will be developed, but when: “It’s inevitable. Just like oil and gas exploration and production, which began on land, then went near-shore and eventually ever further and deeper offshore. Mining shows the same tendency.”
“It’s like dredging at great depths,” says Jan Frumau, managing director of Seatools, near Rotterdam. At a meeting of the Dutch Shipbuilders Society this Thursday, Frumau will deliver a lecture on deep-sea mining. He envisions a fleet of heavy-duty, remotely operated vehicles (ROV’s) operating on the ocean floor, nibbling off the SMS deposits. The electrically powered equipment needs to be extremely tough to operate reliably at 200 bars of hydrostatic pressure. Bringing equipment from the seafloor to the surface and back for repair will take several hours.
Last year professors Van Rhee and Rene Huijsmans (3mE), together with a number of maritime and dredging companies, presented a research proposal at technology fund, STW, called Seaflore, which aimed to develop systems and methods for deep-sea mining, especially targeted at rare-earth metals that are alarmingly becoming scarce.
However, the proposal was rejected because STW felt there was no ‘problem-owner’; that is, a Dutch industry prospecting into deep-sea mining and in need of new technology. True, the professors say, but we do have, close to Rotterdam, a large concentration of leading companies in dredging, offshore lifting and construction, such as Boskalis, Heerema, Fugro and IHC-Merwede, to name a few. IHC-Merwede is starting up a new business unit to support mining industries venturing into deep-sea mining.
Despite STW’s rejection, a number of research projects have been set up at TU Delft. One PhD student will study the vertical hydraulic transport of mine tailings to the surface, while another will work on haptic feedback for underwater robots in Professor Frans van der Helm’s (3mE) STW-supported programme, H-Haptic.
Together with Wageningen University’s research institute Imares, and a team of international researchers, Prof. van Rhee would also like to set up a research project exploring the environmental impact. Environmentalists have expressed concern about the impact of mining activities on deep-sea aquatic life, yet very little is known about the deep sea ecology.
Frumau, on deep-sea mining, Thursday, 19 May at 17:00, Griendencollege, Sliedrecht
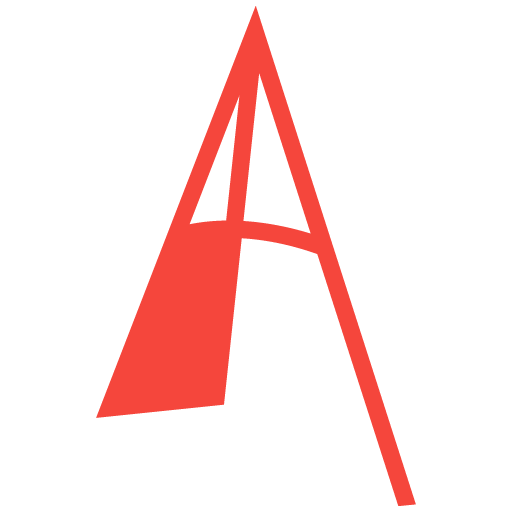
Comments are closed.