The new field of synthetic biology sits in between huge promises of 21st century sustainable production of food, fuels & materials versus tinkered solutions. “We need to develop technology standards.”
An early morning crowd gathered in front of De Veste theatre last Monday morning. Inside, a group of impeccably dressed students, grey suits and orange ties, hosted some 250 visitors to the Life Science symposium on ‘Synthetic Biology’. After two years of preparation, the symposium committee of the LIFE study association (life science and technology) was about to present their line-up of internationally renowned experts in this new field of science that is said to ‘redefine life’.
So what is this ‘synthetic biology’?, chairman Professor Cees Dekker (head of bionanoscience at Applied Sciences) asked the speakers’ forum at the end of the day. “It’s biology turning into an engineering science”, said Professor Martin Fussenegger from ETH Zürich. “It’s using biobricks from nature to build novel combinations”, said Dr Dirk Stemerdink from the Rathenau institute. “It’s like pornography”, said Dr Drew Endy from Stanford University, “you know it when you see it.”
Some see synthetic biology as an enhanced form of genetic engineering. Professor Eriko Takano, who lectures ‘synthetic microbiology’ at the university of Groningen, works along this line. By sequencing the genome of bacteria in search of antibiotic substances she discovered a ‘treasure dome’ of 20 to 50 antibiotic gene clusters per genome. She collects and shuffles these antibiotic building blocks to arrive at new antibiotic compounds against which no bacterial resistance exists yet. “We’re producing natural compounds that don’t exist in nature”, the professor explains. “By changing modules around, you get an enormous variety in synthesised antibiotics.” Professor Takano works with DSM to bring new antibiotics to the market.
But synthetic biology is more than just sophisticated genetic modification. The Swiss Professor Martin Fussenegger (ETH) for example developed an artificial cell to inseminate cows at the right moment. “Insemination of cows is very important for the milk production, which is the basis for all the Swiss chocolate and cheese”, the professor explained only half jokingly. The artificial cell, a cellulose sulphate capsule containing bull’s sperm, is put into a cow’s vagina. It just sits there until it senses the lutenising hormone (LH) rising. The membrane then shrivels, releasing the sperm at exactly the right time of the cow’s ovulation. “It works better than a farmer staring into the cow’s eyes”, explained Fussenegger, who told the audience his group is developing the same technology for human use as well.
Inventive and entertaining as this new technology may be, it’s time for some fundamentals first, argued Dr Drew Endy from Stanford University. As an example he told how much effort it had cost to develop a switchable bit in bacterial DNA. His PhD student ‘poor Jerome’ had succeeded, but only after three years and 7500 assays. “The trouble is: the technology base is missing for successful development”, said Endy. “There is an over-selection in bio-engineering for immediate answers. Therefore not enough effort is put into developing tools and standards.” Most applications were “carelessly engineered” said Endy and “careless made parts can give rise to ‘bonus behaviour’.” Despite parts lists at iGEM’s partsregistry.org and Stanford’s Biofab initiative, universal building blocks are still far away, argues Endy who pleads for a more systematic and fundamental approach.
It’s the private company Amyris (near San Francisco) who took that systematic approach the furthest. Dr Kirsten Benjamin, who leads the microbial physiology group there, sketched the highly automated screening procedure called Automated Strain Engineering (ASE) which is a combination of molecular biology, robotised high trough-put screening (HTS) and software development. Thus DNA-sequences are tested on their phenotypical effects and the results are stored in a growing library that now contains some 20.000 parts. In this way, Amyris develops some 800 yeast strains per week.
The irony is that such data mining projects are better off when publicly funded. Not only because they’re often too expensive for a single company to finance (Amyris lost 60 percent of it’s share value over the last year) but also because the data are not accessible to other researchers. Endy: “Sustained financing is needed over a long time if we’re to realise the enormous potentials of synthetic biology.”
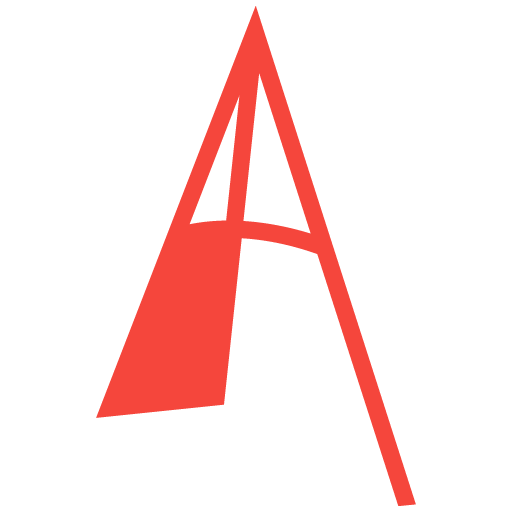
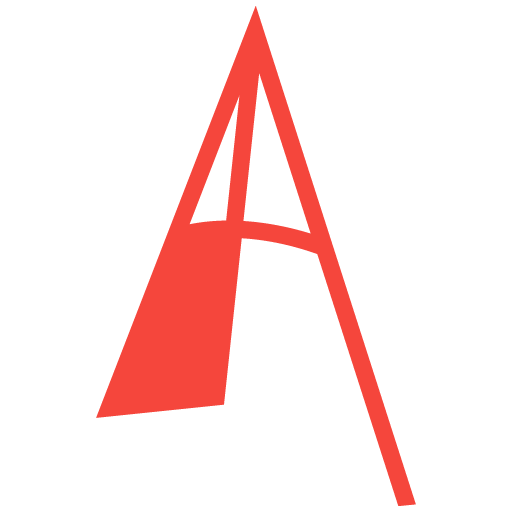
Comments are closed.